Quantum computing is an advanced computer technology that utilizes quantum mechanics principles for performing complex computational tasks. It has the potential to solve problems that are beyond the capabilities of conventional computers.
With the ability to leverage quantum bits or qubits, which can exist in multiple states simultaneously, quantum computers offer the promise of exponentially faster calculations and breakthroughs in areas such as cryptography, optimization, and pharmaceutical research. As the field of quantum computing continues to evolve, researchers and industry leaders are exploring its applications and working towards overcoming the existing challenges to make quantum computing more accessible and practical for various domains.
Quantum computing is revolutionizing the technological landscape, pushing the boundaries of what was once thought possible. With its ability to process vast amounts of data simultaneously, quantum computers are poised to transform industries such as finance, healthcare, and cybersecurity. The potential applications are staggering, from optimizing complex algorithms to solving previously unsolvable problems. Quantum computing is paving the way for advancements in artificial intelligence, drug discovery, and climate modeling. As researchers continue to unlock the power of quantum mechanics, the future of technology looks brighter than ever. Embrace the quantum revolution and prepare for a new era of innovation.
We will delve into the concepts, advantages, challenges, and potential applications of quantum computing.
Understanding Quantum Computing: Advancing Technological Frontiers
Quantum computing is revolutionizing technology by pushing boundaries and enabling new possibilities. Understand the intricacies of this emerging field and its potential to transform industries and solve complex problems.
Quantum Computing: Advancing Technological Frontiers
Quantum computing is an emerging field that has the potential to revolutionize technology as we know it. By harnessing the power of quantum mechanics, this cutting-edge technology offers exciting possibilities for solving complex problems that are currently beyond the reach of traditional computers.
In this section, we will explore the concept of quantum computing, its key components, and the current state of research and development in the field. So, let’s dive in!
The Concept Of Quantum Computing And Its Potential Impact:
- Quantum computing leverages the principles of quantum mechanics, which is the branch of physics that describes the behavior of matter and energy at the smallest scales.
- Unlike classical computers that use bits to represent information as binary digits (0s and 1s), quantum computers use quantum bits or qubits. Qubits can exist in multiple states simultaneously, thanks to a property called superposition, allowing for parallel processing and exponentially increased computational power.
- Quantum computing has the potential to revolutionize fields such as cryptography, drug discovery, optimization problems, machine learning, and weather forecasting.
- The development of quantum computers could significantly impact industries ranging from finance and healthcare to logistics and energy, opening up new avenues for innovation and discovery.
Exploring The Key Components Of Quantum Computing Systems:
- Qubits: The fundamental building block of quantum computers, qubits are the quantum equivalent of classical bits. They can exist in multiple states simultaneously, allowing for quantum parallelism and enabling complex computations.
- Quantum Gates: These are the fundamental operations that manipulate qubits, similar to how classical gates process bits. Quantum gates perform operations like superposition, entanglement, and measurement on qubits, allowing for quantum computation.
- Quantum Circuits: Quantum circuits are composed of interconnected quantum gates. They control the flow of information and quantum operations, resembling the circuitry of classical computers.
- Quantum Error Correction: Quantum computers are highly susceptible to errors caused by decoherence and noise. Quantum error correction techniques aim to mitigate and correct these errors, thereby improving the reliability and stability of quantum computations.
The Current State Of Quantum Computing Research And Development:
- Quantum computing is still in its infancy, but significant progress has been made in recent years. Researchers and companies worldwide are actively working on developing practical quantum computers.
- Quantum supremacy, the point at which a quantum computer outperforms the most powerful classical computers for certain tasks, has been achieved by a few leading organizations.
- However, many challenges remain, including improving qubit coherence, reducing error rates, and scaling up quantum systems.
- Major tech companies such as IBM, Google, Microsoft, and startups like IonQ and Rigetti Computing are driving research and development in the field.
- Governments and institutions worldwide are investing heavily in quantum research, realizing its potential to reshape various sectors and maintain competitiveness in the digital age.
Quantum computing holds immense promise for advancing technological frontiers. Its unprecedented computational power and ability to tackle complex problems could transform numerous industries. As research and development progress, we can anticipate exciting breakthroughs and a future that harnesses the true potential of quantum computing.
Unleashing Quantum Mechanics: The Foundation Of Quantum Computing
Unleashing the foundation of quantum computing, discover the power of quantum mechanics in revolutionizing the future of computational technology. Explore the intricacies of quantum computing and its potential to solve complex problems with unprecedented speed and efficiency.
Quantum computing is a cutting-edge field that holds immense potential for revolutionizing the way we approach complex computations. At its core, quantum computing is built upon the principles of quantum mechanics, a branch of physics that deals with the behavior of matter and energy at the smallest scales imaginable.
In this blog post, we will delve into the foundational aspects of quantum mechanics and explore how these principles enable computations that go beyond the limits of classical computing. Here, we will discuss the role of superposition and entanglement, two fascinating concepts that underpin the power of quantum computing.
Delving Into The Principles Of Quantum Mechanics
- Quantum mechanics is a branch of physics that describes the behavior of particles and systems at the quantum level.
- The principles of quantum mechanics challenge classical notions of determinism and introduce probabilistic interpretations.
- Quantum mechanics encompasses various key concepts, including wave-particle duality, Heisenberg’s uncertainty principle, and the concept of quantized energy levels.
How Quantum Mechanics Enables Computations Beyond Classical Limits
- Quantum mechanics allows for the creation and manipulation of quantum bits, or qubits, which possess unique properties.
- Unlike classical bits that can only represent 0 or 1, qubits can exist in a superposition of states, occupying multiple states simultaneously.
- By utilizing superposition, quantum computers can process massive amounts of information simultaneously, leading to exponential computational speedups.
- Another key aspect of quantum mechanics is entanglement, which enables correlations between qubits that defy classical explanations.
- Entanglement allows for the creation of highly connected quantum systems, enhancing the computational power and capabilities of quantum computers.
The Role Of Superposition And Entanglement In Quantum Computing
- Superposition enables qubits to exist in multiple states simultaneously, exponentially increasing computational possibilities.
- Qubits can be in a combination of two states, such as 0 and 1, thanks to superposition, allowing multiple calculations to be performed in parallel.
- Entanglement allows two or more qubits to become correlated in such a way that their states become interconnected, regardless of the physical distance between them.
- Through entanglement, quantum computers can perform complex calculations faster and more efficiently than classical computers.
- Superposition and entanglement are the cornerstones of quantum computing, providing the potential to solve computational problems that are currently intractable for classical computers.
The principles of quantum mechanics form the foundation of quantum computing. By harnessing superposition and entanglement, quantum computers can tackle computational challenges that are beyond the scope of classical computers. Understanding these quantum mechanical phenomena is crucial for unlocking the extraordinary potential of quantum computing in various fields, including cryptography, optimization, and drug discovery.
As research and development in the field continue to advance, the future of quantum computing looks incredibly promising.
Quantum Bits (Qubits): Harnessing The Power Of Quantum Information
Harness the power of quantum information with quantum bits (qubits) as a key component of quantum computing. Easily manipulate data in a new dimension of computing technology.
Quantum Computing: Harnessing The Power Of Quantum Information
Quantum computing is revolutionizing the world of technology with its incredible potential to solve complex problems that are beyond the capabilities of classical computers. At the heart of this groundbreaking technology are quantum bits or qubits. Let’s dive into the concept of qubits and understand their unique properties.
Explaining The Concept Of Qubits And Their Unique Properties:
- Qubits are the fundamental building blocks of quantum computers, analogous to classical bits in traditional computers.
- Unlike classical bits, which can be either 0 or 1, qubits can exist in a superposition of states, representing both 0 and 1 simultaneously. This is due to the principle of quantum superposition, which allows qubits to process vast amounts of information simultaneously.
- Another intriguing property of qubits is quantum entanglement, where two or more qubits become correlated in such a way that the state of one qubit influences the state of another, regardless of the physical distance between them. This property enables quantum computers to perform parallel calculations and achieve remarkable computational power.
Differentiating Between Classical Bits And Qubits:
- Classical bits are the fundamental units of information in classical computers. They can represent only two states: 0 or 1, which are physically realized as ‘off’ or ‘on’ states, respectively.
- In contrast, qubits have the unique ability to be in a superposition of 0 and 1 simultaneously. This allows quantum computers to perform computations on multiple possibilities simultaneously, exponentially increasing their computing power when compared to classical computers.
- The superposition and entanglement properties of qubits set them apart from classical bits, making quantum computing a potential game-changer in various fields such as cryptography, optimization, and machine learning.
Various Implementations Of Qubits In Quantum Computing Systems:
- Several physical systems can be used to realize qubits, each with its own advantages and challenges. Some of the most promising implementations include:
- Superconducting qubits: These are tiny circuits made of superconducting materials that can carry quantum information. They rely on the unique behavior of superconductors at extremely low temperatures to maintain stable quantum states.
- Trapped-ion qubits: This implementation involves trapping ions in a vacuum chamber and manipulating their internal energy levels to store and process quantum information. Trapped ions have long coherence times, making them suitable for quantum computation.
- Topological qubits: These qubits rely on topological properties of exotic quantum states of matter to store and manipulate information. By exploiting the non-local aspects of these states, errors caused by environmental noise can be minimized.
- Photonic qubits: Photons, the particles of light, can also serve as qubits. They can be manipulated using various techniques such as waveguide circuits and interferometers. Photonic qubits are particularly attractive for their ability to transmit quantum information over long distances through optical fibers.
Implementations of qubits continue to evolve, and researchers are exploring new approaches to overcome existing challenges and scale up quantum computing systems. The future holds immense potential for harnessing the power of qubits and unleashing the true power of quantum information processing.
That wraps up our exploration of qubits and their role in quantum computing. Stay tuned for more exciting insights into the fascinating world of quantum technology.
Quantum Computing Architectures: Building The Foundation For Advanced Computations
Quantum computing architectures lay the groundwork for advanced computations, revolutionizing the capabilities of computer systems. With their potential to solve complex problems more efficiently, quantum computers are set to transform various industries and pave the way for groundbreaking discoveries.
Quantum computing has emerged as a groundbreaking field that holds the potential to revolutionize the way we solve complex computational problems. The success of quantum computing lies in the unique architectures that enable the processing of information using quantum bits, or qubits, which have properties that allow for exponential computational power.
In this section, we will explore the different quantum computing architectures, the significance of quantum gates in computational processes, and the crucial role of quantum error correction in overcoming the challenge of decoherence.
Comparing Different Quantum Computing Architectures:
- Gate-based quantum computing: This architecture utilizes quantum gates to manipulate and process qubits. Quantum gates are the basic building blocks of quantum circuits that enable various computational operations. This architecture strives to create a universal quantum computer capable of solving a wide range of problems.
- Annealing-based quantum computing: Instead of using quantum gates, annealing-based quantum computers rely on a different approach called quantum annealing. This architecture is better suited for solving optimization problems and is particularly effective in scenarios where finding the global minimum is crucial.
- Topological quantum computing: This architecture utilizes exotic particles called anyons to encode and process information. Anyons have properties that make them resistant to decoherence, making this approach particularly promising for building fault-tolerant quantum computers.
- Optical quantum computing: Optical quantum computing relies on manipulating photons as qubits. This architecture offers advantages such as fast processing and long coherence times, but challenges in creating scalable and stable integrated components still need to be addressed.
Quantum Gates And Their Significance In Computational Processes:
- Single-qubit gates: These gates operate on a single qubit and can perform rotations or flips. They are essential for creating superpositions and manipulating the state of individual qubits.
- Two-qubit gates: Two-qubit gates facilitate interactions between pairs of qubits, enabling the creation of entanglement. They play a crucial role in quantum algorithms that rely on entanglement for intricate computations.
- Universal gate sets: A universal gate set is a collection of gates that can be combined to represent any quantum operation. Creating a universal gate set is vital in the pursuit of building a universal quantum computer capable of solving a wide range of problems.
Quantum Error Correction: Overcoming The Decoherence Challenge:
- Decoherence and quantum error: Quantum systems are inherently susceptible to errors caused by various sources of noise and disturbance from the environment. Decoherence refers to the loss of coherence in qubits, which can corrupt the computational process and hinder reliable results.
- Quantum error correction codes: Quantum error correction codes act as a safeguard against errors and decoherence. These codes redundantly encode quantum information, enabling the detection and correction of errors without causing a complete loss of computational data.
- Fault-tolerant quantum computing: Fault-tolerant quantum computing aims to build systems capable of performing reliable computations even in the presence of errors. By implementing quantum error correction techniques, these architectures can mitigate the negative effects of errors and enhance the overall stability and accuracy of quantum computations.
The development of various quantum computing architectures, the significance of quantum gates, and the implementation of quantum error correction techniques are all crucial steps in building the foundation for advanced computations. As researchers and engineers continue to refine and innovate in these areas, the potential of quantum computing to solve currently intractable problems becomes increasingly tangible.
Quantum Algorithms: Transforming Problem Solving
Quantum algorithms have revolutionized problem-solving methods in the field of quantum computing, offering a transformative approach to tackling complex challenges. These algorithms leverage the power of quantum mechanics to provide innovative and efficient solutions that were previously unimaginable.
Understanding The Fundamentals Of Quantum Algorithms
The realm of quantum computing holds immense potential for revolutionizing problem-solving methodologies. Quantum algorithms, the building blocks of quantum computing, offer unprecedented capabilities that have the power to tackle complex problems with remarkable efficiency. To grasp the significance of quantum algorithms, it is essential to explore their fundamentals:
- Superposition and entanglement: Quantum algorithms exploit the principles of superposition and entanglement, enabling qubits (quantum bits) to exist in multiple states simultaneously. This allows quantum computers to explore a vast number of possibilities simultaneously, accelerating problem-solving processes.
- Quantum gates: Similar to classical computing’s logic gates, quantum algorithms utilize quantum gates to manipulate qubits, altering their states and performing calculations. Key quantum gates include the Hadamard gate, Pauli-X gate, and CNOT gate.
- Quantum parallelism: Quantum algorithms leverage quantum parallelism to simultaneously process multiple inputs and explore potential solutions concurrently. This characteristic enables an exponential speedup compared to classical computing for certain problems.
Prominent Quantum Algorithms And Their Applications
A multitude of quantum algorithms have been devised to address specific problem domains and deliver computational advantages that surpass classical computing’s limitations. Some notable quantum algorithms and their applications include:
- Grover’s algorithm: This algorithm accelerates the search process in unsorted databases, providing quadratic speedup compared to classical methods. Grover’s algorithm finds applications in cryptography, optimization, and data analysis.
- Shor’s algorithm: Shor’s algorithm is instrumental in factoring large numbers exponentially faster than classical algorithms. Its potential application in breaking cryptographic codes poses a significant advancement in the field of cybersecurity.
- Quantum machine learning algorithms: Various quantum machine learning algorithms, such as the quantum support vector machine and the quantum k-means clustering algorithm, hold promise for enhancing computational efficiency in analyzing large datasets and pattern recognition tasks.
- Quantum simulation algorithms: Quantum simulation algorithms simulate quantum systems more efficiently than classical computers. They find applications in modeling chemical reactions, optimizing material properties, and investigating the behavior of complex physical systems.
The Potential Impact Of Quantum Algorithms On Various Industries
Quantum algorithms have the potential to revolutionize problem-solving across a range of industries, transforming computational capabilities and enabling breakthrough advancements. The impact of quantum algorithms can be witnessed in:
- Pharmaceutical and drug discovery: Quantum algorithms can accelerate the discovery and development of new drugs by simulating molecular interactions and identifying potent compounds with greater precision.
- Financial industry: Quantum algorithms are poised to transform portfolio optimization, risk analysis, and option pricing, ultimately enhancing investment strategies and financial decision-making processes.
- Supply chain management: Quantum algorithms offer solutions to optimize complex supply chains, improving logistics, inventory management, and route optimization, resulting in cost savings and efficiency gains.
- Artificial intelligence: Quantum algorithms can enhance machine learning models by processing larger datasets more efficiently, enabling faster training and more accurate predictions.
- Cryptocurrency and blockchain: Quantum algorithms have the potential to disrupt blockchain technology by potentially undermining cryptographic mechanisms used in digital currencies and smart contracts.
The impact of quantum algorithms extends beyond these industries, with possibilities yet to be explored. As researchers continue to uncover new quantum algorithms and refine existing ones, the potential for transformative advancements across diverse fields continues to grow. Quantum computing’s promising future hinges upon harnessing the power of these algorithms and pushing the boundaries of what is achievable in computing and problem-solving.
Quantum Computing Challenges And Future Prospects
Quantum computing poses unprecedented challenges and holds immense future prospects in revolutionizing technology. With its ability to process vast amounts of data simultaneously, quantum computing has the potential to solve complex problems that are currently beyond the reach of traditional computers.
The field of quantum computing is advancing at a rapid pace, presenting us with both exciting opportunities and significant challenges. In this section, we will explore the limitations and hurdles faced by quantum computing and delve into the future prospects and potential breakthroughs that lie ahead.
Addressing The Limitations And Challenges In Quantum Computing
- Lack of stability: Quantum systems are highly sensitive to external disturbances, making it difficult to maintain stability and coherence for an extended period.
- Error correction: Quantum bits, or qubits, are prone to errors due to decoherence and environmental noise. Developing robust error correction mechanisms is crucial for reliable quantum computation.
- Scalability: Scaling quantum systems to accommodate larger numbers of qubits remains a significant challenge. The more qubits, the greater the complexity in maintaining coherence and synchronization.
- Complex algorithms: Designing algorithms that harness the full power of quantum computing is a daunting task. Developing efficient algorithms for various computational problems is an ongoing area of research.
- Resource-intensive: Quantum computing requires substantial resources, including specialized hardware, advanced cooling systems, and highly controlled environments. Overcoming these resource limitations is essential for widespread adoption.
Quantum Computing In The Era Of Artificial Intelligence And Machine Learning
- Enhanced optimization: Quantum computing holds the potential to solve complex optimization problems more efficiently than classical computers. This capability has significant implications for artificial intelligence and machine learning algorithms, enabling improved performance in areas such as data analysis, pattern recognition, and recommendation systems.
- Quantum machine learning: The intersection of quantum computing and machine learning opens new avenues for advanced data analysis and predictive modeling. Quantum machine learning algorithms leverage quantum properties, such as quantum superposition and entanglement, to enhance the speed and accuracy of computations.
- Quantum simulations: Quantum computers can simulate quantum systems more effectively than classical computers, enabling the exploration of quantum phenomena at a level not previously achievable. This ability to simulate molecular structures and quantum materials has potential applications in drug discovery, material design, and other scientific endeavors.
The Future Prospects And Potential Breakthroughs In Quantum Computing
- Fault-tolerant quantum computing: Overcoming the challenges of decoherence and errors is crucial to realizing fault-tolerant quantum computing. Advances in error correction codes, fault-tolerant architectures, and qubit technologies are paving the way for practical quantum computers that can perform complex computations reliably.
- Quantum supremacy: Quantum supremacy refers to the point where a quantum computer outperforms classical computers in solving certain problems. Achieving quantum supremacy would mark a significant milestone, demonstrating the unique capabilities of quantum computing and its potential to revolutionize various industries.
- Quantum internet: Building a quantum internet involves developing secure quantum communication networks, enabling the transmission of quantum information over long distances. This could enhance encryption techniques, enable secure quantum key distribution, and facilitate quantum cloud computing.
- Quantum-inspired classical computing: Even before practical quantum computers are fully realized, quantum-inspired algorithms and techniques are making their mark in classical computing. These approaches aim to harness certain characteristics of quantum computing to enhance classical algorithms, leading to improved performance in various computational tasks.
As we continue to push the boundaries of quantum computing, addressing the limitations and challenges while exploring new avenues and breakthroughs, the field holds immense promise. The marriage of quantum computing with artificial intelligence, machine learning, and other technologies opens up a world of possibilities, transforming industries and shaping the future of computing.
Quantum Computing And Cryptography: Reinventing Security
Discover the future of cybersecurity with the groundbreaking potential of quantum computing. This revolutionary technology is set to redefine security measures and revolutionize the way we protect sensitive information.
In the fast-paced digital world, where data is constantly being transmitted and stored, ensuring its security is of utmost importance. Quantum computing is revolutionizing the field of cryptography and promising to reinvent the way we safeguard our confidential information. Let’s delve deeper into the implications of quantum computing on encryption algorithms, advancements in quantum-resistant cryptography, and securing data in the quantum era.
The Implications Of Quantum Computing On Encryption Algorithms:
- Encryption algorithms are the backbone of modern-day security, protecting sensitive data from prying eyes. However, the advent of quantum computers poses a significant threat to these algorithms.
- Quantum computers leverage quantum bits, or qubits, which have the ability to process vast amounts of data simultaneously, exponentially increasing computational power.
- Traditional encryption algorithms, such as RSA and ECC, rely on the difficulty of factoring large prime numbers or solving the discrete logarithm problem. Quantum computers have the potential to quickly solve these problems, rendering these algorithms vulnerable.
- The development of powerful quantum computers could potentially break current encryption schemes, jeopardizing the confidentiality of sensitive information stored in databases or transmitted over networks.
Advancements In Quantum-Resistant Cryptography:
- Acknowledging the imminent threat posed by quantum computers, researchers are actively working on developing quantum-resistant cryptography.
- Quantum-resistant cryptography aims to design encryption algorithms that can withstand attacks from both classical and quantum computers.
- Lattice-based, code-based, multivariate, and hash-based cryptographic protocols are some of the promising approaches being explored.
- These new cryptographic algorithms are based on complex mathematical problems that are believed to be resistant to quantum algorithms.
- Quantum-resistant cryptographic protocols offer a promising solution to ensure the long-term security of sensitive information in the era of quantum computing.
Securing Data In The Quantum Era:
- As the future unfolds, it becomes imperative to prepare for the arrival of quantum computers and the vulnerabilities they may reveal in existing encryption algorithms.
- Organizations and individuals should adapt their security strategies to include quantum-resistant algorithms to safeguard their data.
- Updating encryption protocols and migrating to quantum-resistant algorithms is crucial to ensure the continued confidentiality, integrity, and availability of sensitive information.
- Striking a balance between mitigating the risks posed by quantum computers and maintaining compatibility with existing systems requires careful planning and resources.
- Collaborative efforts from academia, industry, and government entities are essential in addressing the challenges associated with securing data in the quantum era.
Quantum computing carries the potential to revolutionize multiple fields, including cryptography. The implications of quantum computing on encryption algorithms necessitate the development and adoption of quantum-resistant cryptography to ensure the long-term security of sensitive data. By embracing advancements in this area, we can adapt to the ever-evolving threat landscape, protecting vital information in the quantum era.
Quantum Simulation: Revolutionizing Scientific Discovery
Revolutionize scientific discovery with quantum simulation, harnessing the power of quantum computing for groundbreaking advancements. Unveil new insights and overcome complex challenges with this game-changing technology.
The Role Of Quantum Simulation In Solving Complex Scientific Problems
Quantum simulation is a groundbreaking field within the realm of quantum computing that has the potential to revolutionize scientific discovery. By utilizing the unique properties of quantum systems, scientists can simulate complex systems and tackle problems that were previously deemed intractable.
Here is a closer look at the role of quantum simulation in solving complex scientific problems:
- Quantum simulation allows scientists to examine the behavior of quantum systems, such as molecules or materials, in a controlled environment. This enables them to gain valuable insights into the properties and interactions of these systems, which would be impossible with classical computers.
- As a powerful tool in the study of quantum mechanics, quantum simulation provides a means to simulate and explore phenomena that are typically challenging to observe directly. It allows scientists to study intricate quantum phenomena, including entanglement and superposition, on a larger scale.
- One of the key advantages of quantum simulation is its ability to accelerate the understanding of chemical reactions, material properties, and physical phenomena. By modeling complex systems, scientists can develop new materials, optimize chemical processes, and gain deeper insights into fundamental physical theories.
- Quantum simulation has widespread applications in fields like chemistry, physics, and material science. For example, it can help in predicting and designing new drugs, understanding molecular interactions, developing more efficient energy materials, and exploring exotic states of matter.
Applications Of Quantum Simulation In Chemistry, Physics, And Material Science
Quantum simulation finds its applications across various scientific disciplines, generating new possibilities for research and innovation. Here are some specific applications of quantum simulation in chemistry, physics, and material science:
- Chemistry: Quantum simulation allows for the accurate prediction of molecular properties, such as energy levels, reaction pathways, and stability. This capability can drastically speed up the process of drug discovery, as simulations can identify promising drug candidates based on their molecular interactions.
- Physics: Quantum simulation aids in understanding and modeling complex physical systems that exhibit quantum behavior. It can shed light on phenomena such as quantum phase transitions, magnetism, and superconductivity, advancing our comprehension of the underlying principles governing the universe.
- Material Science: Quantum simulation plays a vital role in the design and synthesis of novel materials with desirable characteristics. By simulating the behavior of atoms and molecules, scientists can predict the properties of materials and explore their potential applications in electronics, energy storage, and more.
Accelerating Scientific Advancements Through Quantum Simulation
Quantum simulation holds immense potential to accelerate scientific advancements and push the boundaries of human knowledge. By leveraging the power of quantum computers, researchers can make significant progress in their respective fields. Here are some ways in which quantum simulation facilitates scientific advancements:
- Quantum simulation allows scientists to simulate and analyze systems that are technologically inaccessible, such as complex biological molecules or high-temperature superconductors. These simulations can provide groundbreaking insights into their functionalities and enable the development of new technologies.
- By enabling the study of larger and more complex systems, quantum simulation provides a deeper understanding of fundamental scientific principles. This understanding can lead to the discovery of new theories, the development of innovative solutions, and ultimately, the advancement of scientific knowledge as a whole.
- Quantum simulation holds the promise of revolutionizing industries such as pharmaceuticals, materials science, and energy. Its ability to model and optimize chemical reactions, predict material properties, and explore quantum effects opens up new avenues for innovation and technological advancements.
Quantum simulation offers a paradigm shift in scientific research by providing a powerful tool to tackle complex and previously unsolvable problems. Its applications in chemistry, physics, and material science hold the potential to transform industries and accelerate scientific advancements. By harnessing the capabilities of quantum computers, scientists can unlock unimaginable possibilities and propel scientific discovery to unprecedented heights.
Quantum Computing In Optimization And Machine Learning
Quantum computing offers promising advancements in optimization and machine learning, revolutionizing how we solve complex problems and improve decision-making processes. Its immense computational power and ability to handle vast amounts of data open up new possibilities for industries across the board.
Leveraging Quantum Computing For Optimization Problems
- Quantum computing offers new possibilities for solving complex optimization problems more efficiently and accurately than traditional methods.
- By harnessing the principles of quantum mechanics, quantum computers can explore multiple potential solutions simultaneously, thus enabling faster optimization processes.
- The ability to concurrently evaluate different solutions through quantum states known as qubits allows for enhanced exploration of vast search spaces.
- Quantum optimization algorithms, such as the Quantum Approximate Optimization Algorithm (QAOA) and the Variational Quantum Eigensolver (VQE), demonstrate promising potential in solving various optimization problems.
- These algorithms leverage the power of quantum computing to find the most optimal solution by minimizing or maximizing objective functions.
Quantum Machine Learning Algorithms And Their Potential Applications
- Quantum machine learning has emerged as a rapidly evolving field that combines the power of quantum computing and machine learning techniques.
- Quantum algorithms, such as the Quantum Support Vector Machine (QSVM) and Quantum Neural Networks (QNN), hold remarkable promise for tackling complex machine learning problems.
- QSVM aims to classify data points by leveraging quantum algorithms, and it has the potential to outperform classical machine learning methods in specific cases.
- QNNs, on the other hand, enhance the capabilities of neural networks by incorporating quantum properties such as superposition and entanglement.
- Quantum machine learning algorithms have the potential to revolutionize various domains, including healthcare, finance, logistics, and more, by enabling faster data analysis and more accurate predictions.
Enhancing Efficiency And Performance In Various Industries Through Quantum Computing
- Quantum computing has the potential to significantly enhance efficiency and performance across diverse industries.
- In the healthcare sector, quantum computing can accelerate drug discovery and analysis by simulating complex biological systems more effectively.
- The financial industry can benefit from quantum computing’s ability to solve complex optimization problems related to portfolio management and risk assessment.
- Supply chain optimization, another crucial aspect in various industries, can be improved through quantum algorithms that can optimize routes, inventory management, and logistics.
- Quantum machine learning can boost fraud detection systems by quickly identifying patterns and anomalies in large sets of data.
- Furthermore, quantum computing can revolutionize cryptography and cybersecurity, enabling more robust encryption algorithms and secure communication protocols.
Quantum computing presents exciting opportunities for optimization and machine learning. Leveraging quantum algorithms can enhance performance, solve complex optimization problems, and revolutionize various industries. As this emerging technology continues to evolve, its potential applications and impact will only continue to expand.
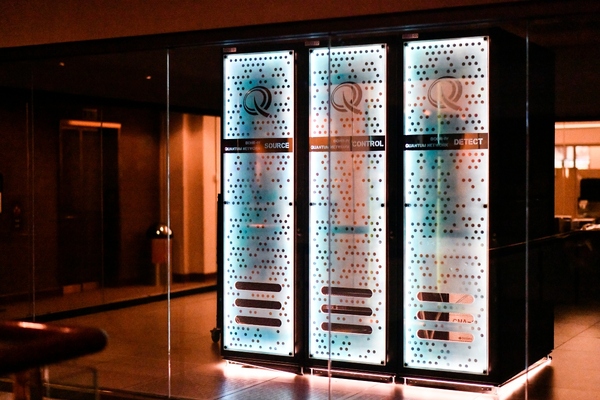
Credit: www.smartcitiesworld.net
Frequently Asked Questions For Quantum Computing
What Does Quantum Computing Actually Do?
Quantum computing is a powerful technology that solves complex problems faster by using quantum bits.
What Is Quantum Computing In Simple Terms?
Quantum computing simplifies complex tasks by using quantum bits, or qubits, to process information more efficiently.
What Does Elon Musk Say About Quantum Computing?
Elon Musk believes quantum computing has the potential to greatly advance technology.
Does Quantum Computers Exist Yet?
Yes, quantum computers exist. They are powerful machines that use quantum mechanics for computing.
What is quantum computing in emerging technology?
Quantum computing is an swiftly advancing technology that utilizes the principles of quantum mechanics to address challenges that exceed the computational capabilities of classical computers.
Conclusion
Quantum computing is a revolutionary technology that holds immense potential for solving complex problems and advancing various industries. With its ability to process massive amounts of data simultaneously, quantum computers promise to revolutionize fields such as cryptography, drug discovery, optimization, and artificial intelligence.
While still in its infancy, the progress made in quantum computing is remarkable, and we can expect further advancements that will lead to practical applications in the near future. The rise of quantum computing brings with it both opportunities and challenges.
As organizations invest in research and development, collaborations between academia and industry will play a significant role in driving breakthroughs in this field. Moreover, it is crucial to ensure that quantum computing is accessible and beneficial to all, promoting inclusivity and diversity within the industry.
Governments must also focus on policies and regulations to address the ethical and security concerns associated with this technology. Quantum computing has the potential to reshape our world in profound ways. By harnessing the power of quantum mechanics, this emerging field opens new doors for innovation and problem-solving.
To truly unlock the transformative power of quantum computing, it will require collaboration, investment, and a forward-thinking mindset. The future of computing is quantum, and its impact on our lives will be nothing short of revolutionary.
More - American Airlines: Discover the Exciting Journey of a Leading Airline